- Forum Supporters
-
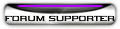
- Elite Member
- Posts: 16,074
- Location: Beltsville, MD
- Logged
https://www.thefreelibrary.com/Influence+of+crankcase+oil+properties+on+low-speed+pre-ignition...-a0478973890Quote
Influence of crankcase oil properties on low-speed pre-ignition encountered in a highly-boosted gasoline direct injection engine.
Author: Teng, Ho; Luo, Xuwei; Hu, Tingjun; Miao, Ruigang; Wu, Min; Chen, Bin; Zeng, Fanhua
Article Type: Report
Date: Nov 1, 2016
Words: 5910
Publication: SAE International Journal of Fuels and Lubricants
ISSN: 1946-3952
INTRODUCTION
[FIGURE 1 OMITTED]
For turbo-charged gasoline direction injection (TGDI) engines with homogeneous mixture combustion, pre-ignition (PI) is frequently encountered, typically at speeds < 2500 rpm and brake mean effective pressures (BMEP) [greater than or equal to] 15 bar. This abnormal combustion is known as low-speed pre-ignition (LSPI). Since PI occurs much earlier than the spark timing for normal combustions, it often leads to severe knock combustion. This abnormal combustion may be characterized with three stages: early-stage combustion triggered by PI, rapid combustion, and severe knock combustion with huge pressure fluctuations as shown in Figure 1. Since the pressure fluctuations during the knock combustion are on the same order of magnitude for the peak firing pressures (PFP) in normal combustions, the PI induced abnormal combustion is also termed as the super knock.
[FIGURE 2 OMITTED]
The cause for LSPI is widely believed to be due to the liquid particles of the crankcase oil entering the engine cylinder [1, 2, 3, 4, 5, 6, 7, 8, 9]. For TGDI engines, the oil enters the cylinder mainly in two ways: as the oil transport through the piston-liner clearance and as the oil loading in the blowby recirculation or the oil carryover. Figure 2 illustrates the oil transport mechanism proposed by Yilmaz [10]: the oil entering the engine cylinder through the piston-liner clearance in three ways, i.e., oil throw-off by the piston motion, the oil mist in the reverse gas flow when the cylinder pressure becomes lower than that in the ring grooves, and evaporation of the oil wetted the cylinder wall. The oil throw off and the oil mist in the reverse gas are related to the piston motion and thus may be combined as the oil transport. According to the test results of Yilmaz, the oil transport increases with the engine speed; at a given engine speed, the oil transport decreases with the engine load as a result of increase in oil evaporation from the cylinder wall [10]. This suggests that the oil transport may not be a unique characteristic for the low-speed and high-load operations where LSPI is encountered.
[FIGURE 3 OMITTED]
For highly boosted TGDI engines, the blowby recirculation reaches its maximum in the LSPI zone on the engine speed-load map [11]. Due to a high level of fuel dilution of the crankcase oil in the LSPI zone [12], the crankcase oil becomes highly volatile and, as a result, considerable liquid oil particles are carried by the blowby recirculation and burned in the engine cylinder as part of the oil consumption. After being cooled at the intercooler, small liquid oil particles may coalesce and form large and more viscous liquid oil particles. Figure 3 shows a phenomenon of hydrocarbon fouling or carbon deposits on the back and stem of the intake valves due to the liquid oil particles in the blowby recirculation for a 1.5L TGDI engine tested by the present authors. Considerable carbon deposits were built up on the backs and stems of the intake valves after the engine was operated at low speeds and high loads for a certain period of time. As the liquid oil particles landed on the intake valves and intake ports, some large and viscous liquid oil particles in the blowby recirculation could also land on the combustion chamber roof during scavenging, which is used as a method for boosting the low speed torques in almost all TGDI engines for [11,12]. Based on the blowby flow rates in the scavenging zone and the characteristics of the liquid oil particles in the blowby recirculation, the number of liquid oil particles entering the engine cylinder as the oil carryover is estimated on orders of [10.sup.7]~[10.sup.8]/cycle for each cylinder [11]. Due to the cooling in the intercooler, the liquid oil particles entering the engine cylinder become less volatile and thus more easily land on the combustion chamber roof with an assist of the air short circuiting flow from the intake ports to the exhaust ports during scavenging. If they are not fully vaporized in the compression stroke, then these liquid oil particles could become the triggers for LSPI.
The influence of the additives in the oil has been studied by many investigators [e.g., 13, 14, 15, 16, 17, 18, 19, 20]. Most of these investigations were focused on the oil-soluble metals added into the oil as the detergents. According to these studies, the impacts on LSPI of the oil additives can be classified as promoters, quenchers, or neutral (no effect). Based on our tests on three different highly boosted TGDI engines (1.5L/1.8L/2.0L) all lubricated with 5W-30 synthetic oil, LSPI is seldom encountered in a new engine with fresh oil, but it is unavoidable for the engines operated for a long period of time, especially with the contaminated crankcase oil and the contaminated intake system. This indicates that the engine condition and the levels of the oil as well as the intake system contaminations have a significant impact on LSPI. In a previous SAE paper [12], we investigated the effect on LSPI of the fuel dilution of the crankcase oil. The aim of this investigation is to understand the influence on LSPI of the crankcase oil with different SAE viscosity grades. Six different market available engine oils were tested with the same engine at the same load point. The oils tested covering SAE 0W-20, 0W-30, 0W-40, 5W-20, 5W-30 and 5W-40 from two different international oil suppliers.
TGDI ENGINE UNDER STUDY
The engine under study is an inline 4-cylinder 1.5L TGDI engine fueled with RON93 gasoline. The basic engine parameters are presented in Table 1. The engine is equipped with a high-efficiency, waste-gated turbo charger and dual cam-phasers allowing both intake and exhaust cams to shift up to 60 deg-CA with respect to their home positions independently. The engine is highly boosted and has a flat torque curve with low-end speed at 1500 rpm and high-end speed at 4600 rpm. Figure 4 shows the engine torque and power curves normalized with their peak values. The side-mounted fuel injector has 6 orifices, with the maximum injection pressure being 150 bar. The fuel spray targets on the piston top are illustrated in Figure 5.
[FIGURE 4 OMITTED]
[FIGURE 5 OMITTED]
The base engine oil is SAE 5W-30 synthetic oil with high temperature and high shear performance. Selected physical properties given in the oil specification are presented in Table 2. Figure 6 shows the distillation curve for the RON93 gasoline used in the engine tests. The oil temperature range for the engine operations is shown in the shaded area for a reference. It is seen that for oil temperatures greater than 140[degrees]C, up to 25% of the gasoline entering the oil may remain in the oil in case of fuel dilution, which is unavoidable in TGDI engines.
[FIGURE 6 OMITTED]
CONDITION FOR LIQUID OIL PARTICLES IN CYLINDER TO BECOME AN IGNITER
Figure 7 illustrates a process for a liquid oil particle in the engine cylinder to ignite the air-fuel mixture. Flash point of the oil is the temperature at which the oil gives off vapors that can be ignited with a flame held over the oil if the oxygen required by the flame is available. Flash point can be used as a measure for the oil volatility [21]. Fire point of the oil is defined as the lowest oil temperature at which the oil vapor produced enables supporting combustion once being ignited. In comparison to gasoline, the engine lubrication oil is a fluid with high boiling point and high auto ignition temperature, with the values for these two characteristic temperatures being very close. Flash point, fire point and boiling point are properties for the liquid oil, but the auto ignition temperature is a property of a combustible gaseous mixture. Figure 8 shows the values of fire point, flash point and viscosity at 100[degrees]C for different SAE viscosity grade oils reported in the literature. Note that flash point and fire point for the engine oils are their high-temperature properties, and thus for two-grade SAE oils these properties are similar to those of the corresponding summer-grade oils. In general, the higher viscosity grade the SAE oils, the lower the oil volatility, and the higher the flash and fire points.
Figure 9 shows the estimated probability for the auto ignition and mass of evaporation at different temperatures for 5W-30 synthetic engine oil [22], where the red line is the auto ignition and the dotted blue line is the distillation curve for synthetic 5W-30 oil. It is seen that the auto ignition is governed by the most volatile species in the oil, i.e., the species with lower boiling points.
[FIGURE 7 OMITTED]
[FIGURE 8 OMITTED]
[FIGURE 9 OMITTED]
[FIGURE 10 OMITTED]
With the characteristics shown in Figure 9, it is difficult for the liquid oil particles in the engine cylinder to become an igniter because the oil mist could be vaporized rapidly and the temperature of the liquid oil particles on the walls of the cylinder and combustion chamber may never reach 300[degrees]C due to the water cooling. However, the crankcase oil properties for highly boosted TGDI engines can be altered with fuel dilution, which can be up to 10% or greater due to condensation of the fuel vapor on the cylinder wall, dispersed liquid fuel drops in contact with the cylinder wall, or impingement of the fuel sprays on the piston top [12]. At low engine speeds and high loads, the level of the fuel dilution is high because of more fuel entering the oil as a result of longer fuel spray penetrations due to high fuel demands at low piston speeds, and a low rate of the gasoline evaporation from the oil at relatively low crankcase oil temperatures. Thus, a large fraction of the heavy species in the gasoline entered the oil can stay in it. The auto ignition temperatures as well as representative species in gasoline are presented in Figure 10, where the red line and dotted blue line plot respectively the auto ignition temperatures and contents of the species in the gasoline. The heavy species in gasoline are those with lower auto ignition temperatures than that for the undiluted oil whose auto ignition temperature typically falls in a range between 300 and 350[degrees]C as shown in Figure 9. The ignitability of the diluted oil is governed primarily by that of the most volatile species in the oil, i.e., the gasoline species with low boiling points and low auto ignition temperatures in the diluted oil.
Figure 11 shows impacts of the gasoline content on the flash point (blue line and square points) and the fire point (red line and triangle points) of the engine oil (5W-30). The flash point and fire point were measured following the standards ASTM D3828-12a (method B) and ASTM D92-12b, respectively. It is seen that the flash point and fire point decrease with increase in the gasoline content. The minimum auto ignition temperature of the diluted oil is determined by the most volatile species in the diluted oil--the heavy species of gasoline in the oil, the auto ignition temperatures of which are close to those of diesel fuel. Fuel dilution causes decreases in the temperatures characterizing volatility, flammability and ignitability of the liquid oil particles entering the engine cylinder, making them have a high tendency for self ignition in the cylinder condition at the late stage of the compression stroke.
[FIGURE 11 OMITTED]
[FIGURE 12 OMITTED]
The relationship between the auto ignition temperature of the liquid oil particles entered in the engine cylinder and the frequency of LSPI was investigated experimentally by Takeuchi et al. [23]. They found that the frequency of LSPI increased with lowering the auto ignition temperature of the oil. Based on their data, a relationship between the LSPI frequency and the auto ignition temperatures of the engine oil is plotted in Figure 12. The correlation shown in Figure 12 indicates that there is a strong link between fuel dilution and LSPI.
LSPI CHARACTERISTICS FOR BASE ENGINE OIL
Behavior of LSPI for the base engine oil (5W-30 synthetic) was investigated experimentally at the low-end maximum torque at 1500 rpm (for short, the low end torque), where LSPI was encountered most frequently. In the tests, the engine was operated with the stoichiometric mixture and the engine-out coolant temperature was controlled at 90[degrees]C. LSPI was noticed to occur highly randomly among the engine cylinders, and was with different patterns [11]. The most representative pattern was that the LSPI events (cycles with PFP up to 180 bar) occurred in a train with one event followed by a normal combustion cycle (with PFP lower than 85 bar) alternatively, as shown in Figure 13. For the particular test shown in Figure 13, the abnormal combustion took place in Cylinder 3. The number of LSPI events in the train varied from just a couple of events to more than 10 events, depending on the levels of the dirtiness in the combustion chamber roof and walls of the intake ports.
[FIGURE 13 OMITTED]
[FIGURE 14 OMITTED]
Figure 14 shows the p-V diagrams for normal and abnormal combustions corresponding to the first LSPI event in Figure 13. PFP for the abnormal combustion in Cylinder 3 occurred much earlier than the rest cylinders with normal combustions, and the value of PFP for Cylinder 3 is much greater than those for the other cylinders. Figure 15 compares the mass of fuel burned (MFB) for the super knock combustion in the first LSPI event in Cylinder 3 (the dash line on the left) with that for the normal combustion cycle for the same cylinder just before the first PI event taking place (solid line on the right). Three differences are apparent: 1) the ignition in the cycle with PI is about 24 degCA earlier than that of the normal combustion cycle, as suggested by the first 5% mass of fuel burned (MFB05); 2) in the second half combustion, represented by the 50% mass of fuel burned (MFB50) to the 90% mass of fuel burned (MFB90), the abnormal combustion is much faster than the normal combustion; 3) the location of PFP for the normal combustion cycle is at about 80% mass of fuel burned (MFB80) but that for the abnormal combustion is pretty much at the onset of the super knock. The fuel mass involved in the super-knock combustion is generally less than 10% as shown in Figure 15.
It is not possible to eliminate the LSPI triggers from the engine cylinder; however, intensities of the super knock can be mitigated through weakening the conditions favoring PI in the scavenging zone. It is known from engine combustion control practice that mixture enrichment has two effects: 1) it increases the ignition delay due to slowing down the ignition kinetics and requiring higher ignition energy as a result of decrease in the oxygen concentration; 2) it accelerates the flame speed as long as the excess air coefficient X less than the value corresponding to the maximum flame speed ([lambda] [approximately equal to] 0.75 for turbo-charged gasoline engines). Increasing the ignition delay retards the start of combustion ignited by the liquid oil particles, making the crank angle for PI move to the top dead center (TDC), and acceleration in the flame speed reduces the end gas involved in the knock combustion, both of which mitigate knock intensities.
[FIGURE 15 OMITTED]
[FIGURE 16 OMITTED]
Figure 16 compares cylinder pressure traces for two LSPI events with the engine being operated with a stoichiometric mixture and an enriched mixture with X equal to 0.75 respectively. The engine was operated under the low-end torque with the engine coolant temperature being 90[degrees]C. It is seen that the mixture enrichment did not prevent LSPI but it did mitigate the intensities of knock. Note that for the case with the enriched mixture, LSPI occurred in Cylinder 4, not Cylinder 3 (the pressure trace for Cylinder 2 was not recorded due to a malfunction transducer). The terms of LSPI and super knock should not be used interchangeably because if the flame speed is sufficiently high, the pre-ignition may not lead to knock combustion. Note that pre-ignition stresses on that the mixture is ignited by a difference source before the spark ignition, while knock is an abnormal combustion phenomenon occurring at the late stage of the combustion, only related to a small portion of the mixture known as the end gas whose temperature reaches that for auto ignition before the flame front reaches it. The faster the flame speed, the less the end gas involved in the knock combustion, and the weaker the knock intensity.
LSPI CHARACTERISTICS FOR DIFFERENT OILS
Procedure for LSPI Testing
It was noticed that LSPI was unavoidable when entering the LSPI zone from low speeds but it rarely occurred when entering the same zone from high speeds, as illustrated in Figure 17, where the zone specified by the red line indicates the loads and speeds at which LSPI events are encountered, and the zone for scavenging is indicated by the green line with double dots. The intake and exhaust manifold pressures for the full load curve are also presented in Figure 17. For the engine under study, there existed reverse flows of the hot exhaust gas from the exhaust ports to the intake ports during the valve overlap at engine speeds greater than 2500 rpm, and this hot reversed flow made some liquid oil particles on the intake ports and intake valve backs vaporized; as a result, the effective number of the liquid oil particles entering the cylinder in the scavenging flow followed became less than that when entering the LSPI zone from low engine speeds. For results in Figure 17, the crank oil was the base engine oil.
[FIGURE 17 OMITTED]
In order to understand the influence of different type of the engine crankcase oil on the characteristics of LSPI, six different market available engine oils were tested with the same engine at the low-end torque load point. The oils tested were market available products from two international oil suppliers, covering SAE 0W-20, 0W-30, 0W-40, 5W-20, 5W-30 and 5W-40, with all of them being synthetic. Viscosities, flash points and fire points for these oils agree well with those shown in Figure 8. The investigations were conducted only on the engine operations with a stoichiometric mixture.
LSPI characteristics for the selected oils were tested following a same 5hr-test procedure, covering 3.5hr operations for the "engine conditioning" and 1.5hr operations for the LSPI test. In the conditioning stage, the engine was operated for 2.5 hrs under high loads with 1 hr at 4000rpm with 70% load and 1.5 hrs at the low-end torque, followed by 1hr at idle. After conditioned, the load point of the engine was switched to the low-end torque for the LSPI test. The low-end torque load point was operated twice for different purposes: the first one was for generating hydrocarbon fouling on the intake ports and intake valves, and the second one was for the LSPI test. The LSPI characteristics were studied only when entering the LSPI zone from low speeds. The test on each type of the engine oil was repeated three times and only the worst case was selected for comparison. A correlation between the cylinder pressures and the knock sensor signals was pre-determined for the engine under study, and a threshold for the overpressure in detecting a LSPI event was set based on PFP values in the normal combustion cycles and the maximum PFP values in the spark knock combustions. When the cylinder pressure reached the threshold, the cylinder pressures for 41 cycles were recorded as one data file, with 20 cycles before and 20 cycles after the first detected overpressure cycle. The number of cycles in one recording was selected based on our observations: the LSPI events occurring in a sequence seldom exceeded 10.
LSPI Characteristics for Different Oils
In order to including the influence of the engine coolant temperature on the characteristics of LSPI, the tests were conducted with the two different engine coolant temperatures: 90[degrees]C and 105[degrees]C, respectively. For all the oils tested, fuel dilution levels and flash points were tested after each of the tests. The fuel dilution levels in the duration of the test procedure are shown in Figure 18. The fuel dilution test was conducted following the standard ASTM D352504 (2010), which represents the 95% probability. The flash points for the diluted oils fell in a range between 87 and 97[degrees]C, with the 0W oils having higher flash points than the 5W oils. These values are about 150[degrees]C lower than the fresh oils [24], suggesting that volatility and ignitability of these oils be influenced considerably by the fuel dilution.
[FIGURE 18 OMITTED]
The results for some oils with poor repeatability of the LSPI events due to unknown reasons were not taken for comparison. Selected test results are presented in Tables 3 to 6. The results indicate that the behavior of LSPI is highly random. As can be seen that 0W oils are not necessary to be better than 5W oils, and there is no clear trend for the influence on LSPI of the oil viscosity or the engine coolant temperature. In our previous tests on the base engine oil [11], the frequency of LSPI was observed to decrease with increasing the coolant temperature. However, in this investigation, the influence of the engine coolant on LSPI was not apparent.
Selected cases for illustrating the random behavior of LSPI are given in the following. Figure 19 shows a LSPI test with the engine lubricated with 0W-30 (supplier-1 product) and the coolant temperature being 105[degrees]C. In this particular data recording, the LSPI event train had 12 events, with 11 of them associated with superknock (cycles with high PFP values). Figure 20 presents the result of a repeating test under the same condition. In this case, only a single LSPI event was detected in a different cylinder, and the preignition did not lead to knock combustion. Figure 21 compares the cylinder pressure trace for the first LSPI event in Figure 19 with that for the isolated LSPI event in Figure 20. In the repeating test, the cylinder pressure fluctuations are negligible as shown in the right chart in Figure 21. Regardless a LSPI event leads to superknock or not, it still should be classified as abnormal combustion because its pressure rising rate after start of combustion and PFP are much greater than those in the normal combustion cycles, and thus engine vibrations will be different from those in the normal combustion cycles. This is also the mechanism for detecting the LSPI events with the engine knock sensor.
[FIGURE 19 OMITTED]
[FIGURE 20 OMITTED]
[FIGURE 21 OMITTED]
[FIGURE 22 OMITTED]
Figure 22 compares the cylinder pressure traces for a LSPI test (left chart) and those for a repeating test (right chart) under the conditions with the engine lubricated with 0W-20 (supplier-2 product) and the oil temperature being 105[degrees]C. In both tests, only an isolate LSPI event was detected. Although occurred in the same cylinder in these two tests, the LSPI event in the repeating test did not lead to super knock as shown in the right chart in Figure 22.
We noticed in this investigation that although LSPI occurred frequently as long as the engine load and speed fell into the LSPI zone, the behavior of LSPI was different and the event often occurred in different cylinders. It was difficult to reproduce the same LSPI pattern in the same cylinder with the engine operated under the same conditions. This suggests that LSPI be triggered in a complicated condition with contributions from many variable parameters, related to the intake flow, the exhaust flow, physical conditions of the liquid oil particles entering the engine cylinder, the number density of the liquid oil particles in the blowby recirculation, the scavenging duration and flow rate, the level of hydrocarbon fouling in the intake system, etc. It is only meaningful to characterize the behavior of LSPI in a statistical sense.
Impact of Hydrocarbon Fouling on Intake Valves and Ports
It was noticed in the tests that LSPI occurred mainly in the scavenging zone on the engine map given in Figure 17. As was aforementioned, in the scavenging zone, hydrocarbon fouling or carbon deposits can be significant on the intake ports as well on the back and stem of the intake valves. Figure 23 shows a LSPI test with the engine oil being 0W-20 (supplier-1 product) and the coolant temperature being 105[degrees]C. In this test, 13 LSPI events (cycles with PFP values about 150 bar) occurred in the event train in Cylinder 4, with all of them leading to superknock. The number of LSPI events in the event train was the highest among all of the LSPI tests that we conducted so far.
[FIGURE 23 OMITTED]
In order to investigate the impact of the hydrocarbon fouling in the intake system on LSPI, the black, sticky, sluge-type hydrocarbon fouling on the inner surface of the outlet pipe of the intercooler as well as on the walls the intake manifold and intake ports was wiped out with towels with removal of the intake manifold assembly from the engine. After this cleaning action, the intake assembly was reinstalled then the test was restarted under the same engine operation conditions. The harder hydrocarbon fouling on the intake valves was not touched being afraid of that the falling offs could enter the engine cylinder. The result of the test with the partially cleaned intake system is presented in Figure 24. It is seen that although the intake system cleaning action did not eliminate LSPI, there were only three LSPI events in the event train and they occurred in Cylinder 1, with Cylinder 4 behaved normally. In all the tests on other oils with the partially cleaned intake system, LSPI occurred rarely, and once occurred the number of the LSPI events in the event train became much less. Due to the random nature of LSPI, it may not be concluded that the triggers for LSPI were originated from the oil loading in the blowby recirculation; however, it can be stated based on our tests that the hydrocarbon fouling in the intake system has a strong influence on the LSPI events in the event train, i.e., how many LSPI events occur in a sequence when LSPI is encountered.
[FIGURE 24 OMITTED]
SUMMARY
An experimental investigation was conducted on the influence of the crankcase oil properties on the engine combustion in the LSPI zone with a highly boosted 1.5L TGDI engine. The engine oils tested covered SAE 0W-20, 0W-30, 0W-40, 5W-20, 5W-30 and 5W-40. In the tests, the fuel dilution levels reached 5.3 to 6%. With these levels of the fuel dilution, the flash points for the diluted oils dropped by about 150[degrees]C, indicating that the properties of the crankcase oil were altered by the fuel dilution. Since the gasoline species were the most volatile and ignitable components in the diluted oils, the volatility and ignitability of the diluted oils differed from those of the fresh oils significantly. There was no clear indication on which SAE oil tested had a stronger influence on LSPI than other oils, either promoting or inhibiting LSPI. This may be due to that the influences on LSPI of the related properties for the different oils might not be as strong as that of the gasoline content in the diluted oils. For any tested oil, it was difficult to reproduce the same LSPI pattern in the same cylinder with the engine operated under the same conditions. This suggests that LSPI be triggered in a complicated condition with contributions from many variable parameters, related to the intake flow, the exhaust flow, physical conditions of the liquid oil particles entering the engine cylinder, number density of the liquid oil particles in the blowby recirculation, the scavenging duration and flow rate, the level of hydrocarbon fouling in the intake system, etc. It may be only meaningful to characterize the behavior of LSPI in a statistical sense.
Ho Teng, Xuwei Luo, Tingjun Hu, Ruigang Miao, Min Wu, Bin Chen, and Fanhua Zeng
Jiangling Motors, Co., Ltd
REFERENCES
[1.] Willand, J., Daniel, M., Montefrancesco, E., Geringer, B., et. al., "Limits on Downsizing in Spark Ignition Engines due to Preignition," MTZ, 70: 56-61, 2009.
[2.] Haenel, P., Seyfried, P., Kleeberg, H., and Tomazic, D., "Systematic Approach to Analyze and Characterize Pre-ignition Events in Turbocharged Direct-injected Gasoline Engines," SAE Technical Paper 2011-01-0343, 2011, doi:10.4271/2011-01-0343.
[3.] Kalghatgi, G.T. and Bradley, D, "Pre-ignition and 'Super-knock' in Turbo-charged Spark-Ignition Engines," International Journal of Engine Research,13(4): 399-414, 2012.
[4.] Welling, O., Moss, J., Williams, J., and Collings, N., "Measuring the Impact of Engine Oils and Fuels on Low-Speed Pre-Ignition in Downsized Engines," SAE Int. J. Fuels Lubr. 7(1):1-8, 2014, doi:10.4271/2014-01-1219.
[5.] Dahnz, C. and Spicher, U., "Irregular Combustion In Supercharged Spark Ignition Engines--Pre-Ignition and Other Phenomena," International Journal of Engine Research, 11: 485-498, 2010, dio:10.1243/14680874JER609.
[6.] Zaccardi, J.-M., Lecompte, M., Duval, L. and Pagot, A., " Preignition in Highly Charged Spark Ignition Engines--Visualisation and Analysis," MTZ, 70: 56-61, 2009.
[7.] Heiss, M. and Lauer, T., "Analysis of Pre-ignition Initiation Mechanisms using a Multi-Cycle CFD-Simulation," International Multidimensional Engine Modeling User's Group Meeting 2014, Detroit, US, April 7, 2014,.
[8.] Heiss, M. and Lauer, T., "Analysis of Particle Separation With Respect to Pre-Igniton in An Si-Engine," 11th World Congress on Computational Mechanics (WCCM XI), Barcelona, Spain, July 20-25, 2014.
[9.] Gunther, M., Uygun, Y., Kremer, F. and Pischinger, S., "Pre-Ignition and Glow-Ignition of Gasoline Biofuels," MTZ, 74:46-53, 2013.
[10.] Yilmaz, E., "Sources and Characteristics of Oil Consumption in a Spark-ignition Engine," MIT PhD thesis, 2003.
[11.] Luo, X., Teng, H., Hu, T., Miao, R. et al., "Mitigating Intensities of Super Knocks Encountered in Highly Boosted Gasoline Direct Injection Engines," SAE Technical Paper 2015-01-0084, 2015, doi:10.4271/201501-0084.
[12.] Hu, T., Teng, H., Luo, X., and Chen, B., "Impact of Fuel Injection on Dilution of Engine Crankcase Oil for Turbocharged Gasoline Direct-Injection Engines," SAE Int. J. Engines 8(3):1107-1116, 2015, doi:10.4271/2015-01-0967.
[13.] Welling, O., Collings, N., Williams, J., and Moss, J., "Impact of Lubricant Composition on Low-speed Pre-Ignition," SAE Technical Paper 2014-01-1213, 2014, doi:10.4271/2014-01-1213.
[14.] Dingle, S., Cairns, A., Zhao, H., Williams, J. et al., "Lubricant Induced Pre-Ignition in an Optical SI Engine," SAE Technical Paper 2014-011222, 2014, doi: 10.4271/2014-01-1222.
[15.] Morikawa, K., Moriyoshi, Y., Kuboyama, T., Yamada, T. et al., "Investigation of Lubricating Oil Properties Effect on Low Speed Pre-Ignition," SAE Technical Paper 2015-01-1870, 2015, doi:10.4271/201501-1870.
[16.] Moriyoshi, Y., Yamada, T., Tsunoda, D., Xie, M. et al., "Numerical Simulation to Understand the Cause and Sequence of LSPI Phenomena and Suggestion of CaO Mechanism in Highly Boosted SI Combustion in Low Speed Range," SAE Technical Paper 2015-01-0755, 2015, doi:10.4271/2015-01-0755.
[17.] Morikawa, K., Moriyoshi, Y., Kuboyama, T., Yamada, T. et al., "Investigation of Lubricating Oil Properties Effect on Low Speed Pre-Ignition," SAE Technical Paper 2015-01-1870, 2015, doi:10.4271/201501-1870.
[18.] Boese, D., Ritchie, A., and Young, A., "Controlling Low-Speed Pre-Ignition in Modern Automotive Equipment: Defining Approaches to and Methods for Analyzing Data in New Studies of Lubricant and Fuel-Related Effects (Part 2)," SAE Technical Paper 2016-01-0716, 2016, doi:10.4271/2016-01-0716.
[19.] Ritchie, A., Boese, D., and Young, A., "Controlling Low-Speed Pre-Ignition in Modern Automotive Equipment Part 3: Identification of Key Additive Component Types and Other Lubricant Composition Effects on Low-Speed Pre-Ignition," SAE Int. J. Engines 9(2):832-840, 2016, doi:10.4271/2016-01-0717.
[20.] Long, Y., Wang, Z., Qi, Y, Xiang, S. et al., "Effect of Oil and Gasoline Properties on Pre-Ignition and Super-Knock in a Thermal Research Engine (TRE) and an Optical Rapid Compression Machine (RCM)," SAE Technical Paper 2016-01-0720, 2016, doi:10.4271/2016-01-0720.
[21.] Ishida, H. and Iwama, A., "Some Critical Discussions on Flash and Fire Points of Liquid Fuels," Fire Safety Science: Proceedings of the First International Symposium, 218-226, 1986.
[22.] Hu, T., Teng, H., Luo, X., Lu, C. et al., "Influence of Fuel Dilution of Crankcase Oil on Ignitability of Oil Particles in a Highly Boosted Gasoline Direct Injection Engine," SAE Technical Paper 2015-01-2811, 2015, doi:10.4271/2015-01-2811.
[23.] Takeuchi, K., Fujimoto, K., Hirano, S., and Yamashita, M., "Investigation of Engine Oil Effect on Abnormal Combustion in Turbocharged Direct Injection--Spark Ignition Engines," SAE Int. J. FuelsLubr. 5(3):1017-1024, 2012, doi:10.4271/2012-01-1615.
[24.] Luo, X., Teng, H., Hu, T., Miao, R. et al., "Mitigating Intensities of Super Knocks Encountered in Highly Boosted Gasoline Direct Injection Engines," SAE Technical Paper 2015-01-0084, 2015, doi:10.4271/201501-0084.
CONTACT INFORMATION
Ho Teng
Chief Engineer in Engines
Jiangling Motors Co., Ltd
Phone: +86 791 8526-7012
hteng1@jmc.com.cn
ACKNOWLEDGMENTS
The authors thank colleagues of the gasoline engine team at JMC for helps during the engine tests on LSPI and Shanghai Oil Gas & Chemicals Testing Center of SGS for conducting the crankcase oil property measurements.
ABBREVIATIONS
BDC--Bottom dead center of piston
BMEP--Brake mean effective pressure
PI--Pre-ignition
LSPI--Low speed pre-ignition
MFB--Mass of fuel burned
MFB05--5% of mass of fuel burned
MFB50--50% of mass of fuel burned
MFB90--90% of mass of fuel burned
PFP--Peak firing pressure
TDC--Top dead center of piston
TGDI--Turbocharged gasoline direct injection
VVT--Variable valve timing
Table 1. Basic parameters for the TGDI engine
under study.
Engine configuration 14
Engine displacement 1.5L
Bore 79 mm
Stroke 76 mm
Compression ratio 9.5:1
Number of valves per cylinder 4
Fuel injection equipment Direct injection
Max injection pressure 150 bar
Injector mounting Side mounted
Fuel RON93 gasoline
Turbo flow control Waste gate
Table 2. Selected properties of engine crankcase oil.
Oil grade 5W-30
Density @ 15[degrees]C [kg/L] 0.852
Viscosity @ 100[degrees]C [cSt] 11.7
Viscosity @ 40[degrees]C [cSt] 67.5
Total base number TBN [mg-KOH/g] 10
Flash point [[degrees]C] 229
Noack volatility @ 250[degrees]C [wt%] < 10
Table 3. Oils from supplier-1, engine coolant temperature
= 90[degrees]C.
SAE grade 0W-30 0W-40 5W-20 5W-30 5W-40
#PI in event train 1 7 2 1 1
#PI w/ superknock 1 6 1 1 0
#PI w/o super knock 0 1 1 0 1
[PFP.sub.max] [bar] 125 155 130 125 115
Table 4. Oils from supplier-1, engine coolant temperature
= 105[degrees]C.
SAE grade 0W-20 0W-30 0W-40 5W-30
#PI in event train 13 12 4 6
#PI w/ superknock 13 11 3 6
#PI w/o superknock 0 1 1 0
[PFP.sub.max] [bar] 155 155 155 155
Table 5. Oils from supplier-2, engine coolant temperature
= 90[degrees]C.
SAE grade 0W-40 5W-20 5W-30 5W-40
#PI in event train 1 1 1 1
#PI w/ superknock 0 1 0 1
#PI w/o superknock 1 0 1 0
[PFP.sub.max] [bar] 115 125 115 150
Table 6. Oils from supplier-2, engine coolant temperature
= 105[degrees]C.
SAE grade 0W-20 0W-30 0W-40 5W-20 5W-30 5W-40
#PI in event train 1 1 8 2 6 3
#PI w/ superknock 1 0 7 1 6 2
#PI w/o superknock 0 1 1 1 0 1
[PFP.sub.max] [bar] 150 110 155 135 155 155
COPYRIGHT 2016 SAE International
Copyright 2016 Gale, Cengage Learning. All rights reserved.
2007 Ford Edge SEL, Powerstop F/R Brake Kit, TXT LED 6000K Lo & Hi Beams, W16W LED Reverse Bulbs, 3BSpec 2.5w Map Lights, 5W Cree rear dome lights, 5W Cree cargo light, DTBL LED Taillights
If tuned: Take note of the strategy code as you return to stock (including 3 bar MAP to 2 bar MAP) -> take car in & get it serviced -> check strategy code when you get car back -> have tuner update your tune if the strategy code has changed -> reload tune -> ENJOY!